Section 4 part a) and b)
a) Units
4.1 use the following units: kilogran (kg), joule (J), metre (m), metre/second (m/s), metre/second2 (m/s2), newton (N), second (s), watt (W).
b) Energy transfer
4.2 describe energy transfers involving the following forms of energy: thermal (heat), light, electrical, sound, kinetic, chemical, nuclear and potential (elastic and gravitational)
some examples we had in class:
- battery connected to a light bulb: chemical-kinetic-light & heat
- candle and a box of matches: kinetic-light & heat
- elastic band and a 'paper pellet': elastic-kinetic
- bowl of breakfast cereal eaten: solar-chemical-kinetic + heat
- plant in a pot by the window: light (solar) - chemical
Piece of equipment
|
Input energy
|
Output energy
| |
Useful
|
Waste
| ||
Mains light bulb
|
Electrical
|
Light
|
Thermal
|
Electric fire
|
Electrical
|
Thermal
|
Light
|
Battery
|
Chemical
|
Electrical
|
Thermal
|
4.3 understand that energy is conserved
Energy is not created nor destroyed, it is just transferred from one form to another.
Thermal energy always flows from a region of higher temperature to a region of lower temperature.
4.4 recall and use the relationship:
efficiency= useful energy output/total energy input
If you use the following equation, remember to add a percentage sign (%):
efficiency= useful energy output/total energy input x 100
If you use the following equation, remember to add a percentage sign (%):
efficiency= useful energy output/total energy input x 100
4.5 describe a variety of everyday and scientific devices and situations, explaining the fate of the input energy in terms of the above relationship, including their representation by Sankey diagrams
Sankey diagrams shows what happens to the input energy and in what proportion it is transferred to various forms. For example:
This is the Sankey diagram for a typical filament lamp.
![]() |
Sankey diagram for a filament lamp |
Ordinary electric lamps contain a thin metal filament that glows when electricity passes through it. However, most of the electrical energy is transferred as heat energy instead of light energy.
The efficiency of the filament lamp is (10 ÷ 100) × 100 = 10%.
This means that 10% of the electrical energy supplied is transferred as light energy (90% is transferred as heat energy).
![]() |
Sankey diagram for a typical energy-saving lamp |
From the diagram, you can see that much less electrical energy is transferred, or 'wasted', as heat energy.
The efficiency of the energy-saving lamp is (75 ÷ 100) × 100 = 75%. This means that 75% of the electrical energy supplied is transferred as light energy (25% is transferred as heat energy).
Note that the efficiency of a device will always be less than 100%.
Relating to specification point 4.3, remember that energy is conserved. It is neither created nor destroyed, just transferred from one form to another or moved. So here energy may be 'wasted', as it isn't the form we want-which is light. But the heat energy from the lamps don't just disappear, they are transferred to the surroundings so sometimes you feel hot sitting under the lamps. And the heat energy spreads out until it becomes difficult to do anything useful with it.

4.6 recall that energy transfer may take place by conduction, convection and radiation

Conduction: is the process of thermal energy transfer without any flow of the material medium. It is from particle to particle (as they move faster, they push on neighbouring particles so they speed up too). (Occurs mainly in solids.)
**This has apparently confused some people, about what 'flow of the material medium' actually means. Sorry about that, I think I took the definitions from a textbook somewhere and didn't read it properly to see if it was easy to interpret. Hm, well, in conduction heat is transferred:
-when atoms vibrate and heat is transferred from one atom to another
-or when free electrons move from the hot end to the cold end (hence metals are good conductors of heat-they have free electrons)
So the material isn't moving, the metal wire (or whatever medium) isn't going anywhere when it conducts heat. Whereas in convection, the water/air molecules are moving and their movement creates convection currents to transfer heat. Remember conduction mainly occurs in solids, they can't move around like fluids (liquids/gases) can.
Hope that makes sense now. :)
**This has apparently confused some people, about what 'flow of the material medium' actually means. Sorry about that, I think I took the definitions from a textbook somewhere and didn't read it properly to see if it was easy to interpret. Hm, well, in conduction heat is transferred:
-when atoms vibrate and heat is transferred from one atom to another
-or when free electrons move from the hot end to the cold end (hence metals are good conductors of heat-they have free electrons)
So the material isn't moving, the metal wire (or whatever medium) isn't going anywhere when it conducts heat. Whereas in convection, the water/air molecules are moving and their movement creates convection currents to transfer heat. Remember conduction mainly occurs in solids, they can't move around like fluids (liquids/gases) can.
Hope that makes sense now. :)
Convection: Occurs in fluids (liquids and gases). It is the transfer of thermal energy by means of currents in a fluid (liquids or gases). E.g. If you were to heat a beaker of water in one spot, the water above the flame expands and thus becomes less dense. So it rises upwards as cooler, denser water around it sinks and displaces (pushed it out of the way) it. So this creates a circulating stream called a convection current. So as the water circulates it transfers the energy to other parts of the beaker.
Radiation: This type of energy transfer doesn't require a medium. The energy is transferred by electromagnetic radiation travelling at the speed of light-it is actually a specific part of this family of electromagnetic waves we are talking about called infrared waves. (Electromagnetic radiation is covered in another post.) So radiation is the continual emission of infrared waves from the surface of all bodies, transmitted without the aid of a medium.
- Dull, dark surfaces absorb infrared radiation faster compared to shiny, white surfaces but they are also better emitters of the radiation, so they actually cool down faster.
- Shiny, bright surfaces reflect the radiation and so absorb less of it.
4.7 describe the role of convection in everyday phenomena
e.g. convection in air-gases--warm air rises as it is displaced by cooler, denser air sinking around it.
Heated by the sun, warm air rises above the equator as it's displaced by cooler, denser air sinking to the North and South.
Result: huge convection currents in the Earth's atmosphere. This causes winds across all oceans/continents.
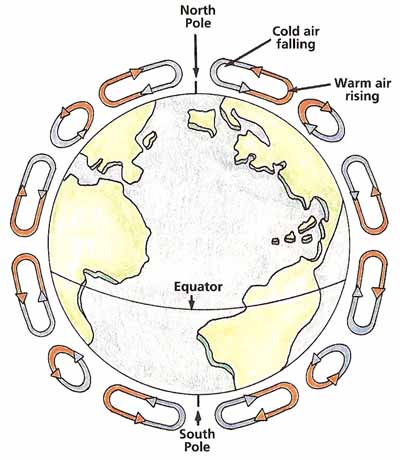
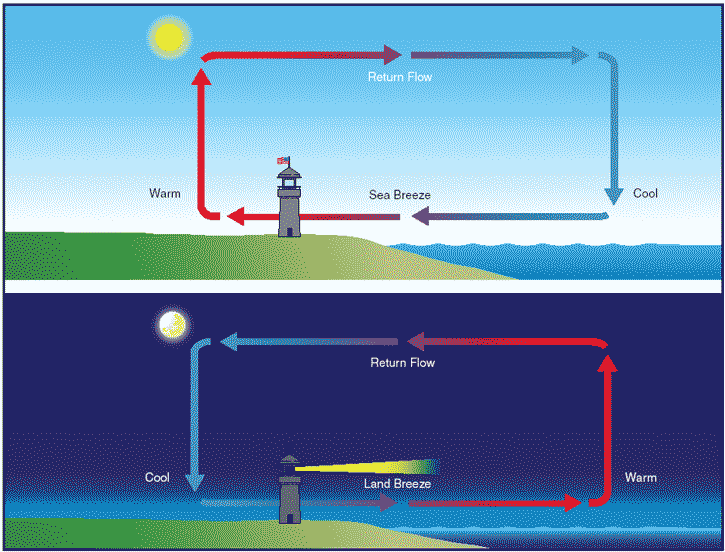
During the day, land heats faster than water, so the air over the land becomes warmer and less dense. It rises and is replaced by cooler, denser air flowing in from over the water. This causes an onshore wind, called a sea breeze. Conversely, at night land cools faster than water, as does the corresponding air.
In this case, the warmer air over the water rises and is replaced by the cooler, denser air from the land, creating an offshore wind called a land breeze.
4.8 describe how insulation is used to reduce energy transfers from buildings and the human body
air is a bad conductor of heat so many insulators contain tiny pockets of trapped air to stop heat being conducted away

House, energy can be lost through + insulation:
- roof: loft and roof insulation
- walls: wall cavity filled with insulator such as polystyrene foam
- draughts: draught excluder on doors and windows
- floor: carpet
- windows: double-glazed windows (has gap where air is trapped between the two panes of glass, which reduce thermal energy transfer through the windows), curtains also help insulate
For the human body, we wear woolen clothes to keep ourselves warm, wool feels warm because it traps a lot of air.
Air is a relatively bad conductor of heat because the particles are much further apart than in a solid. So collisions between particles are much less frequent, thus transfer of kinetic energy to neighbouring particles is much slower.
Whereas in solids, the particles are packed tightly together so if one end is heated the particles vibrate vigorously and collide with neighbouring particles making them vibrate as well. Thus the kinetic energy of the vibrating particles at the hot end is transferred to the neighbouring particles quickly.
Hope this helped. :)